International cooperation is extremely important in high energy science. Only a large collaboration of scientists and universities can create world-wide experiment – like Large Hadron Collider (LHC). National Research Nuclear University «MEPhI» actively supports international cooperations by sending its students all over the world.
In 2015, 4 master and graduate students from department of «Experimental methods of nuclear physics» №11 in the Center of Fundamental Research (CFR) spent several months working on the most important experiments of high energy physics. Our press center asked them to tell their exciting stories.
Sergey Fedotov, graduate:
In 2015 I had an opportunity to participate in activities connected with NA62 experiment in the European Organization for Nuclear Research (CERN) during three months. My work was funded by NRNU MEPhI.
The main goal of NA62 experiment is to study a rare kaon decay (K + → π + νν). Cross section measurements of this process allow to test the theoretical predictions of the Standard Model (SM). Deviations from the theory may indicate the existence of physics beyond the SM. This decay is strongly suppressed, so studies require a large amount of data. Previous results included high errors. We expect to achieve uncertainty of 10% with new NA62 measurements.
My task was connected with developing and testing one of the main part of NA62 detector: Charged-particle hodoscope NewCHOD. I’ve also participated in detector monitoring (so called shifts) during the NA62 operation. It is a time when all detectors are collecting statistics for future physics analysis. My master's thesis was based on my studies during stay in CERN.
During these three months I successfully used the knowledge obtained in NRNU MEPhI and enriched it with the experience of my colleagues in CERN.
My trip had gone very quickly leaving only pleasant memories. I express my deep gratitude to MEPhI, department №11 «Experimental methods of nuclear physics» and in particular to my supervisor prof. Kudenko Yuri Grigorievich for all these positive emotions and invaluable experience.
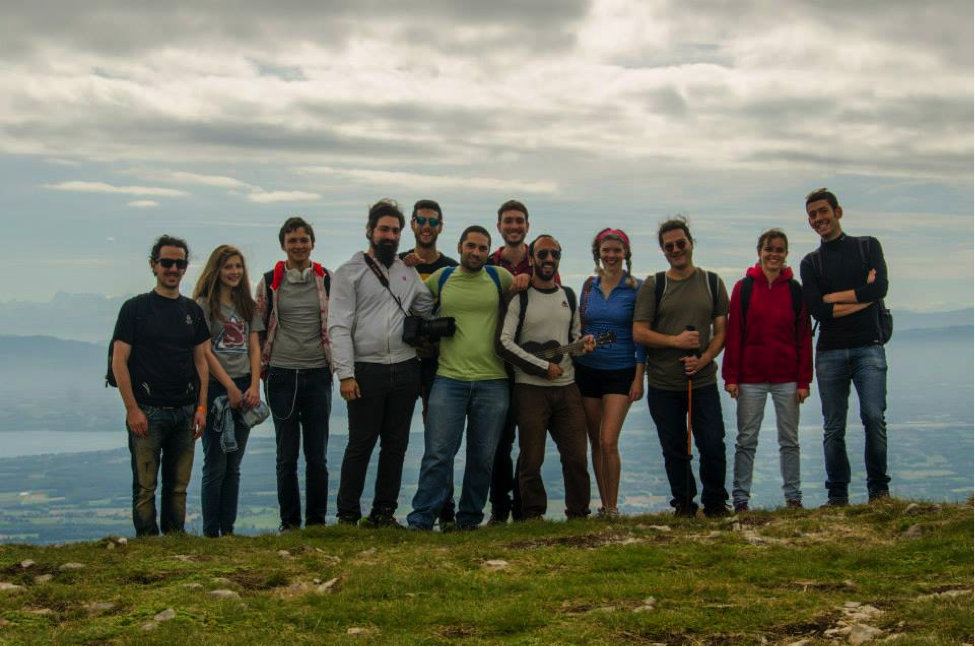
Sergey (third on the left) with NA62 collaboration members.
Alina Kleimenova, student:
When I was at school, I was listening to the news on the radio from the European Organization for Nuclear Research and thinking that it should be a pretty interesting place. I have not ever imagined to find myself there.
However, this summer I had the opportunity to work for the NA62 experiment. My scientific work associated with this experiment began from work with INR RAS research group. It was silicon photomultipliers testing for NewCHOD detector. Тew horizons were opened once I arrived to Geneva. Firstly, I was lucky enough to take part in detector monitoring shifts – it made possible to look at the work of a large experiment from the inside. And secondly, I started analyzing the data obtained in these sessions. My new colleagues provided me a lot of support in my studies.
During my internship I was able to learn how to use available software to write my own programs to evaluate the performance of some detectors and to get skills in processes modelling in the scintillator (NewCHOD is a scintillation hodoscope). I also started working on my master's thesis which is dedicated to search for heavy neutrinos in the kaons decay.
Looking back, I can say that it was great 90 days filled with new interesting acquaintances, excursions, hiking and of course experimental physics. I am very glad I was able to be in such a beautiful place to work for so long.
As it turned out, this was not my last visit to CERN. I took part in collaboration meeting in February, where the next data collecting period and analysis of already accumulated data were discussed.
Maria Antonova, student:
My scientific work is related to the study of muon range detector SMRD characteristics. It based on INR RAS platform, supervising by prof. Kudenko Yuri Grigorievich (in MEPhI) and senior scientist Izmailov Alexander Olegovich (in RAS HEP). SMRD detector is array of scintillation counters placed in ND280 detector magnet of the long-baseline T2K experiment. Its main purpose is to study neutrino oscillations parameters.
This year I participated in the regular collaboration meeting which took place in Tokai, Japan. Meetings are held 4 times a year, but this time it was dedicated to the celebration in honor of the "Breakthrough Prize" laureate Koichiro Nishikawa, who made a great contribution to neutrino physics research.
A journey to the T2K meeting was a great opportunity to talk personally with people involved in the experiment and to learn details of their research. Such meetings incredibly encourage to work in experimental physics.
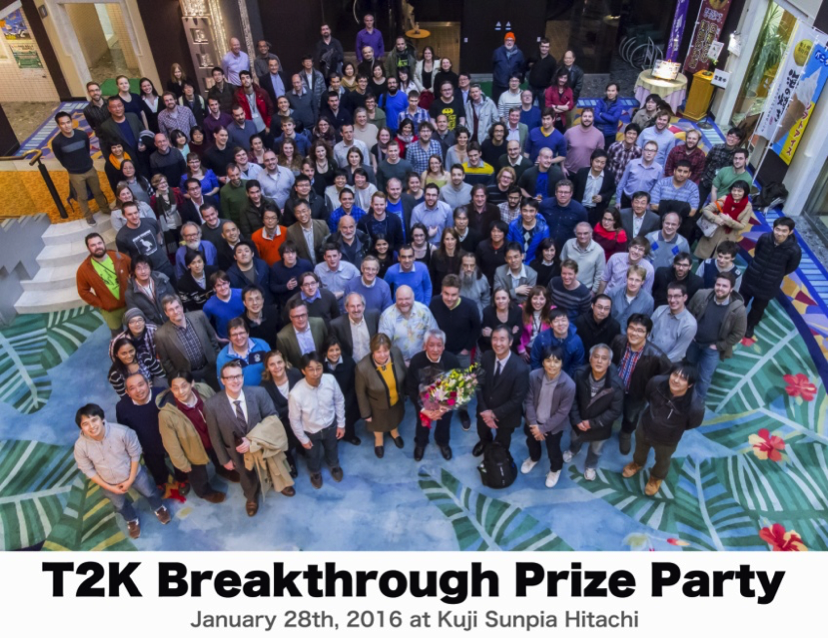
T2K collaboration and Breakthrough Prize laureate Koichiro Nishikawa
Tatiana Ovsiannikova, graduate student:
In the second half of 2015 assembling works for pilot detector of WAGASCI experiment (Water-Grid-Scintillator-Detector) have been started. One of the physics goals of WAGASCI experiment is the measurement of the cross sections ratio between neutrino to water and neutrino to hydrocarbon. It will allow to reduce systematic errors related to the target substance in the far and the near detector of the neutrino accelerator experiment with long-baseline T2K. The second goal is charged current neutrino interaction channels measurements.
WAGASCI pilot detector is a water-filled unit (one of four) of WAGASCI detector central target. One of the INGRID iron-scintillation detector modules will be used as the muon range detector. It will work on the on-axis beam of the J-PARC accelerator.
In October 2015, as a graduate student of the department «Experimental methods of nuclear physics» of NRNU MEPhI CFR I took part in the WAGASCI detector prototype construction in Tokai, Japan, where the accelerator facility J-PARC and the near detector ND280 of T2K experiment are based.
At the moment prototype detector construction is near the completion and physics operation will start in March. Each member of our group has been involved in the manufacturing process of scintillation counters, assembly and various tests. My scientific work is related to the computer simulation, so it was very interesting to construct the real detector with my own hands.
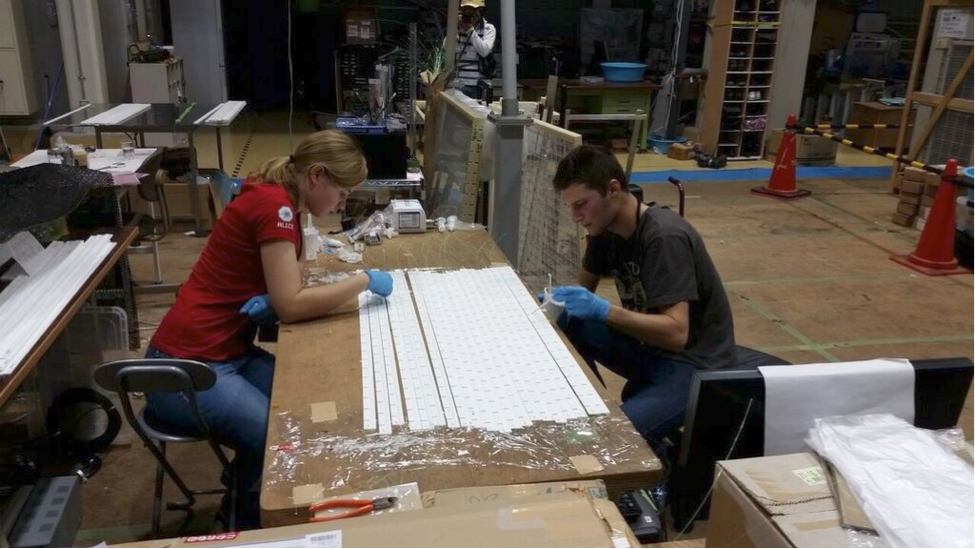
Collaboration members at work (Tatiana on the left)